
Technology
Technical Background
Tethered Membrane Technology
Tethered membranes are lipid bilayers that are used as model cell membranes for a wide range of biophysical and physiological research. SDx manufactures a tethering foundation chemically attached to a thin gold film that acts as an inert base and electrode. A selection of natural or synthetic lipids can then be added to create a stable bilayer that models the properties of cell membranes for the purpose of ion channel, toxin and membrane studies using electrical or fluorescent measures.
There have been many formats developed to support lipid bilayers for use as model membranes (Supported Membranes: Scientific and Practical Applications. E. Sackmann. Science 5 January 1996: 43-48).
SDx tethering technology has the advantages of the stability of the disulphide chemistry, a four nanometre hydrophilic sub-membrane space and a flexibility of lipid composition.
For background see video Introduction to tBLM
Lipid Gold Chemistry
The formation of a t-BLM occurs in four steps as shown in Figure 1.
Figure 1. Formation of a t-BLM.

step 1: gold immersed in ethanol-lipid solution
step 2: lipids reacts with gold and tethers to surface.
step 3: addition of non-tethered lipids in typically ethanol.
step 4: rinse with PBS to form t-BLM.
Figure 2. Tethered lipids

(a) tethered phytanyl bis-tetra-ethyleneglycol

(b) hydroxyterminated-bis-tetra-ethyleneglycol
SDx provides lipid coated gold electrodes (steps 1 and 2). The researcher forms a bilayer with the addition of second layer lipids (steps 3 & 4).
To coat the gold electrodes lipids are chemically bound to the gold substrate using a benzyl disulphide (Figure 2a) which is more stable against oxidation than a simple thiol and also provides optimal spacing of the ethylene glycol linkers (Figure 2b) to eliminate conduction limitation of ionic species within the reservoir region between the BLM and the gold electrode.
A range of slide coatings with different tether densities is available. They are described by Tm(x) where x= the ratio expressed as the % of the tethered fraction that penetrates the bilayer and forms the inner layer leaflet of the t-BLM (see figure 2(a) above), relative to the tethered fraction that terminates in an OH group at the surface of the inner leaflet of the bilayer (see figure 2(b) above), The advantage of a range of % tethers within the inner leaflet of the t-BLM is that it permits experiments to be designed where ion channels or polypeptides can be optimally incorporated into the bilayer.
The average spacing of the t-BLM tether (figure 2(a) above) can be used as an approximate guide to the molecular weight of moieties to be accommodated within the untethered fluid lipid fraction of the t-BLM. The basis of this estimate is a simple volume calculation based on the molecular weight of the membrane associated component and an assumed thickness of 4nm for the t-BLM. All slide coatings ranging from 0 < x < 100 will form bilayers however a trade-off exists between the stability and ionic leakage of the t-BLM and the volume of fluid lipid bilayer available to incorporate additional membrane associated species such as an ion channel.
When designing an experiment we recommend using the highest tether density possible for a particular molecular weight species being incorporated into the t-BLM. The molecular weights are calculated according to this simple geometric model. Of course this molecular weight may only be a fraction of the molecular weight of a whole ion channel or other membrane associated compound under study.
SDx manufactures gold electrodes coated with lipid tethers (step 2 above) stored in purified alcohol for long term stability (tethaPlate). A lipid bilayer can be formed on the tether coated electrode by addition of a lipid mix of choice.
Alternate tether chemistries are available - see Custom Lipids
Forming a Membrane
In preparation for use the gold electrodes supplied are rinsed with ethanol, then dried. A further ethanol solution containing mobile outer layer lipids (figure 3) is added and the slide rinsed with PBS spontaneously forming a t-BLM.
Figure 3. Mobile layer lipid species.
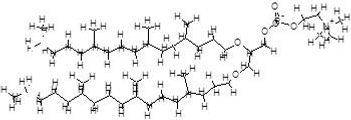
a) diphytanyletherphosphatidylcholine

b) Glycerodiphytanylether
The mole ratio of the two components is 70:30 for compounds 3(a):3(b). The reason for the mixture is to achieve an average area per molecule at the hydrocarbon-aqueous interface that is commensurate with the average area per molecule at the centre of the bilayer. This ratio may require adjustment for different included species within the membrane. This ratio is best determined empirically for any particular included species.
Alternative lipid mixes can be used depending on experimental requirements - see Custom lipids
Measuring Conductance
The SDx tethaPod conductance reader in its most basic operation reports the conductance across a tethered bilayer membrane formed in a tethaPlate. With this conductance measure the properties of the membrane itself or the change in conductance in response to the insertion of ion channels can be studied without a requirement for an in-depth understanding of Impedance Spectroscopy.
Tethered Membranes because of the limited ions available in the reservoir use AC current rather than the standard DC current used in patch clamp equipment. The SDx membranes have a area that is an order of size greater patch clamp and therefore has a seal of a mega Ohm scale rather than the giga Ohm scale of patch clamp. Although single channel measurements are not available the tethered membrane principle provides robust with sensitivity and flexibility of technique.
For more sophisticated electrophysiology studies tethaPlate can be connected to any potentiostat via a tethPatch to offer additional capability.
For AC current both phase and impedance are calculated at each frequency providing a range of metrics to determine the electrical properties of the membrane. These include the admittance at the frequency for minimum phase (YminP: provides membrane conductance), and the admittance at 1kHz (Y1kHz from which the membrane thickness may be determined).
For DC current, millisecond bursts from the potentiostat overcome the limitations of available ions in the reservoir allowing voltage gating studies using a tethaPlate-tethaPatch-potentiostat configuration.
The equivalent electrical circuit of a tethered membrane is shown in Figure 4.
Ions in the saline solution create two capacitors: C1 (~0.5µF/cm2), due to the insulating barrier of the membrane; and C2 (~3.5µF/cm2,) due to ions crowding at the gold surface. A 10 to 50mV swept frequency a.c. excitation in the range 1 to 1000HZ is applied between the gold electrode and a large area gold return electrode on the facing surface of the flow cell cartridge. Current passing through C1 and C2 is measured across the reference resistor R. The voltage across R due to the current flow is amplified in amplifier A and the output supplied via a USB port to a computer for processing and analysis.
Figure 4. Electrically Equivalent Circuit
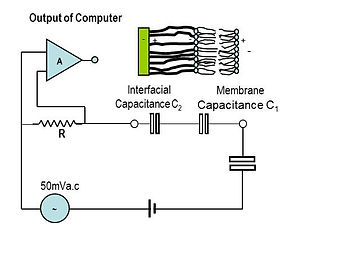
Software packages are provided which further permit a calculation of the kinetics of admittance changes of the membrane when channels are introduced or when ligands are added which block the channel.
Inserting Ion Channel Proteins and Peptides
Ionophore (channel or carrier) incorporation
The incorporation of Ionophores, into a membrane can occur by many mechanisms the major approaches being:
-
Spontaneous insertion for Ionophores such as peptides like Valinomycin or protein ion channels such as the Chloride Intracellular Ion Channel (CLIC), or the bacterial ion channel ?-Haemolysin into a preformed t-BLM.
-
Detergent assisted insertion for Ionophores such as the mechanosensitive channel (MscL). PBS rinsing of coated electrodes exposed to detergent dispersions of MscL forms t-BLMs containing MscL.
-
Proteoliposomal fusion for Ionophores such as the voltage dependent anion channel (VDAC) where the t-BLM forms from liposomes containing the VDAC ion channel.
-
Fusion of Proteoliposomes containing an ion channel with a preformed t-BLM.
In each case empirical optimization of the insertion conditions is advised. The % tethering of the t-BLM may be adjusted to optimize the balance between the stability and seal of the membrane against the the amount of material that may be inserted. In the case of liposomal fusion to form a t-BLM, the fusion process requires >Tm(50) coated slides to stimulate the fusion process from aqueous liposomal dispersion. This may be reduced to lower Tm(x) values by using detergent solutions to increase the fusion rate of the liposomes.